Beyond sustainability: Future-proofing with resilient design strategies
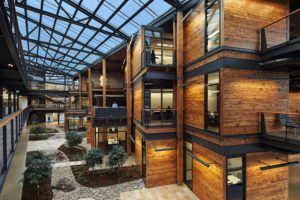
In recent years, increasing climate-related natural disasters have brought the topic of resilient design to the forefront. So-called 100-year events are being redefined to catch up to the realities of an increasingly unstable natural environment. Building design professionals must now consider the many elements shaping the resilience of the built environment.
Where do design professionals start? With the very first elements of a project: client goals as expressed through guiding principles. The clients are the ones making the informed decisions that guide the relationship between aspirational goals, design criteria and the realities of a project budget to accommodate current requirements while anticipating future needs. Client goals are the true shapers of a project’s resilient design approach.
For resiliency goals to be successfully integrated into a project’s aspirations, the design team must help clients understand and properly define their own criteria by identifying major areas of resilient design. In this context, it is the design team’s responsibility to provide the education: What is resiliency? What is the meaning of the different levels of resiliency and how does each level protect the building and its inhabitants for the short and long term? A critical part of this process is addressing expectations regarding “proofing” versus “resistance.” From the viewpoint of the client, how resistant will the building be to earthquakes, floods, hurricanes or fire? Is the client getting the desired level of resilience for the prescribed budget?
These critical conversations provide focus and a clear path forward for choosing the appropriate level of building resiliency.
Seismic resilience
Seismic design can prevent catastrophic building collapse and ensure structures behave in a predictable manner in the event of an earthquake. A baseline measure of resilience can often be achieved in seismic design if the vertical lateral force resisting elements (shear walls and bracing) are arranged in a manner to improve the lateral drift (stiffness) and desired structural dynamic response of the building in a seismic event. In highly active seismic regions, designing to code may be inadequate to prevent permanent structural damage as prescriptive building code requirements are set as minimum requirements to protect life-safety, and not post-event structural integrity. Clients can also choose to pursue performance-based design strategies that push seismic design beyond the code’s prescriptive methods.
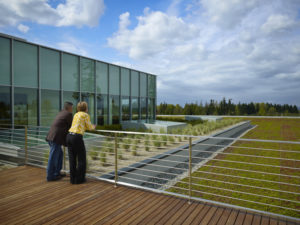
Seismic performance-based design strategies for the Mark, a 48-story, 69,677-m2 (750,000-sf) commercial office and hotel tower in downtown Seattle, Wash., demonstrated that the structure met or exceeded the intended performance of the code with regards to strength, stiffness, ductility, life safety and collapse prevention. This criteria consisted of running seven simulated earthquake ground motions through a non-linear dynamic analysis of the tower to demonstrate all of the building’s components met performance criteria that ensures structural integrity is maintained. The simulation validated that the Mark could withstand a 2,475-year seismic event with a magnitude of 9 on the Richter Scale. This approach led to a better overall understanding of structural seismic performance, and for this project in particular, resulted in enhanced predicted seismic performance above code minimum requirements. By wrapping around the entire building, the perimeter bracing system acts as a closed tube that engages the axial stiffness and strength of all the perimeter steel columns. Slight yielding of the perimeter steel braces and concrete core reinforcing steel is expected, but there would be no loss in integrity of any structural elements or connections.
Among performance-based design strategies, seismic base isolation is one of the most effective methods for controlling vibration and increasing the likelihood of the building withstanding a seismic event. At the Nintendo of America headquarters in Redmond, Wash., resilient design strategies for the accompanying data center included a seismic base isolation and a rotary uninterrupted power supply system.
In addition to structural system performance, other major building systems are called on to remain fully operational after a seismic event. For mission-critical facilities, including government and healthcare buildings, it is imperative to plan for extended durations of infrastructure interruption. Strategies such as seismic base isolation and utility redundancy add an increased level of protection against structural collapse and damage to building systems, while net-zero and net-positive strategies allow operations to continue even when the grid is unavailable.
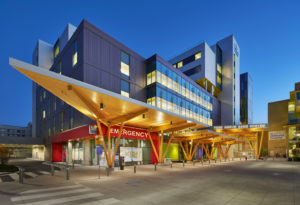
For example, due to the fault line location at the Teck Acute Care Centre, BC Children’s Hospital and BC Women’s Hospital + Health Centre in Vancouver, British Columbia, the facility was designed above minimum local seismic code, and to meet the post-disaster facility requirements of the National Building Code of Canada.
The hospital, which serves some of the region’s most critically ill patients, was designed to stringent energy standards that support both performance goals and resilience requirements. The design provides N+1 level redundancy, ensuring any individual piece of mechanical equipment can be out of service without compromising peak system capacity, and the facility can operate at post-disaster capacity for three full days by using emergency generators, while underground systems for potable water and sewage collection enable its continuous operations. To achieve an absolute energy target, the mechanical design makes use of advanced heat-recovery devices to simultaneously optimize heating and cooling. Air-cooled chiller/heat recovery eliminates the need for water in cooling. Water-saving strategies reduce the total volume of onsite water storage needed to provide 72 hours of domestic water.
Additional design solutions to help maintain operations include conversion of the project’s ambulance canopy into a three-lane mass decontamination shower with zones for undressing, showering and dressing; numerous inpatient rooms with double headwall capacity to provide for surge populations; and six patient wings that can be easily converted into pandemic outbreak control zones. Finally, a conference room is fitted with equipment and storage that anticipates its conversion into an operations hub during an emergency.
Climate resilience
Climate responsive design is a critical component of any resiliency approach. Not only do designers plan for current climate conditions in a given region — wildfires in the mountainous West for example — but they increasingly anticipate and design for future changes in the climate. In addition to the fundamental considerations of passive design, strategies for resiliency include planning for increased temperatures and thermal loads, either in the base design through passive or mechanical means, or incorporating flexibility and adaptability in systems to address in the future.
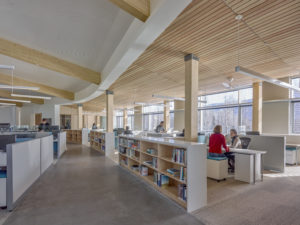
Designed as a 100-year structure, the Rocky Mountain Institute’s Innovation Center in Basalt, Colo., exceeded the requirements for the Leadership in Energy and Environmental Design’s pilot credit for passive survivability and functionality during emergencies, which assesses whether a building can maintain safe thermal conditions for four consecutive days in the event of an extended power outage or loss of heating fuel. With its focus on passive house, net zero and climate responsive elements, the Innovation Center was modeled to operate passively without any energy for heating, ventilation and air-conditioning for an entire year — maintaining the required and expanded comfort zone, even as winter temperatures dipped below -18 C (0 F). The passive structure features a photovoltaic assembly with full battery backup and lightning protection.
While current Colorado state law prohibits onsite water retention and reuse to prevent impacting historical downstream users, designers incorporated an innovative approach to enable net-zero non-potable water use as laws change. Roof stormwater is channeled by the building’s subtle butterfly roofs into a water feature on the southern edge of the building atrium, filtered by a series of swales, and carried to Old Pond, just west of the project site where water rights are owned by the town of Basalt.
Notably, the Innovation Center was built following a regional effort to reconfigure the banks of the adjacent Roaring Fork River to address flooding events that threatened homes and introduced pollution into the ecosystem. The site design enhances this effort, respecting existing Category IV wetlands with a minimum 15-m (50-ft) buffer and restoring additional wetlands onsite. Raising the building out of the 500-year floodplain accommodates spring snowmelt and allows seasonal inundations to continue unimpeded by human impact. The building directs stormwater through natural swales into wetlands, and site pathways use permeable paving.
Social resilience
Resilience can also be measured in social terms. Direct multi-sensory connections to the exterior environment as well as internal connections are critical aspects of a perceived level of safety and security. For example, common spaces in buildings where occupants can gather establish a shared internal sense of community and provide a positive support system for occupants in the event of a disaster.
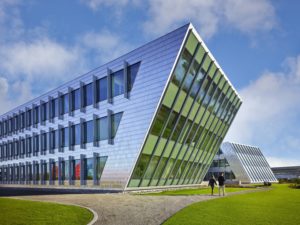
Designed to withstand severe weather events and a potential seismic event, the Federal Center South Building 1202 is a 19,417-m2 (209,000-sf) regional headquarters for the U.S. Army Corps of Engineers in Seattle, Wash. The high-performance design keeps critical operation areas in service during severe weather events while also providing a 21st century workplace environment bathed in natural daylight. The “oxbow” design concept allows the architecture itself to participate in social resilience, providing a unified and flexible open office layout to connect all employees. The Commons — a central area where all conference rooms and shared services are located at the core of the building — compels users to come together and functions as the social hearth. The Commons utilizes 200,000 board feet of timber reclaimed from a warehouse previously located on the site. The timber adds to the interior environment’s aesthetics, enhancing the sense of community and collective identity within the building, while also supporting notions of biophilia.
To prevent progressive collapse, the building utilizes an exposed perimeter steel diagrid structure, providing a visual sense of strength and rigor as expressed in the redundant truss support system for floor framing, should one of the columns be taken out; and an innovative site system of stone columns and earthquake drains reduces the risk of site liquefaction and lateral spreading. The building’s foundation is supported by 49-m (160-ft) steel piles, driven through the sandy soils to the glacial till below. The ground floor consists of a structural slab spanning between the piles, with below-grade piping hung from the floor slab, so even if liquefaction does occur and the grade drops several feet due to a significant seismic event, the building will remain standing, providing occupants with a continued sense of security and stability after a ground-altering seismic event. All of the framing for the exterior window system is structurally reinforced and all the exterior glass is laminated. Critical operation areas are connected to the emergency generator with a tank sized for a seven-day/24-hour fuel supply.
Additionally, Federal Center South integrates active and passive systems, materials, and strategies to achieve aggressive water- and energy-saving requirements without sacrificing comfort or amenity. Energy and water introduced into the building are leveraged to their highest possible use. Conditioned air is delivered to the workspace via air-handling units and exhausted passively through the atrium to high-efficiency heat recovery systems. Available daylight, controlled for glare and unwanted heat gain with a varying degree of frit across the atrium skylight in response to sun exposure, is directed to the office floor plates from two sides, to offset the maximum possible amount of electric lighting.
Conclusion
Sustainability has long been the major driver in designing high-performing buildings. The architecture, engineering, and construction industries have made great progress in designing more sustainable and better performing buildings. Building energy consumption has decreased substantially due to better thermal envelopes, more efficiently looped mechanical and plumbing systems, optimal electrical consumption, use of healthier materials, and public awareness related to energy management.
Resiliency can be viewed as a continuation of this path, elevating sustainable design to an even higher level of performance that minimizes a building’s downtime after an event takes place, and possibly even shielding a structure from the damaging long-term effects of a natural disaster. Resiliency is not simply another layer of a building design. It is the project’s ultimate sustainability.